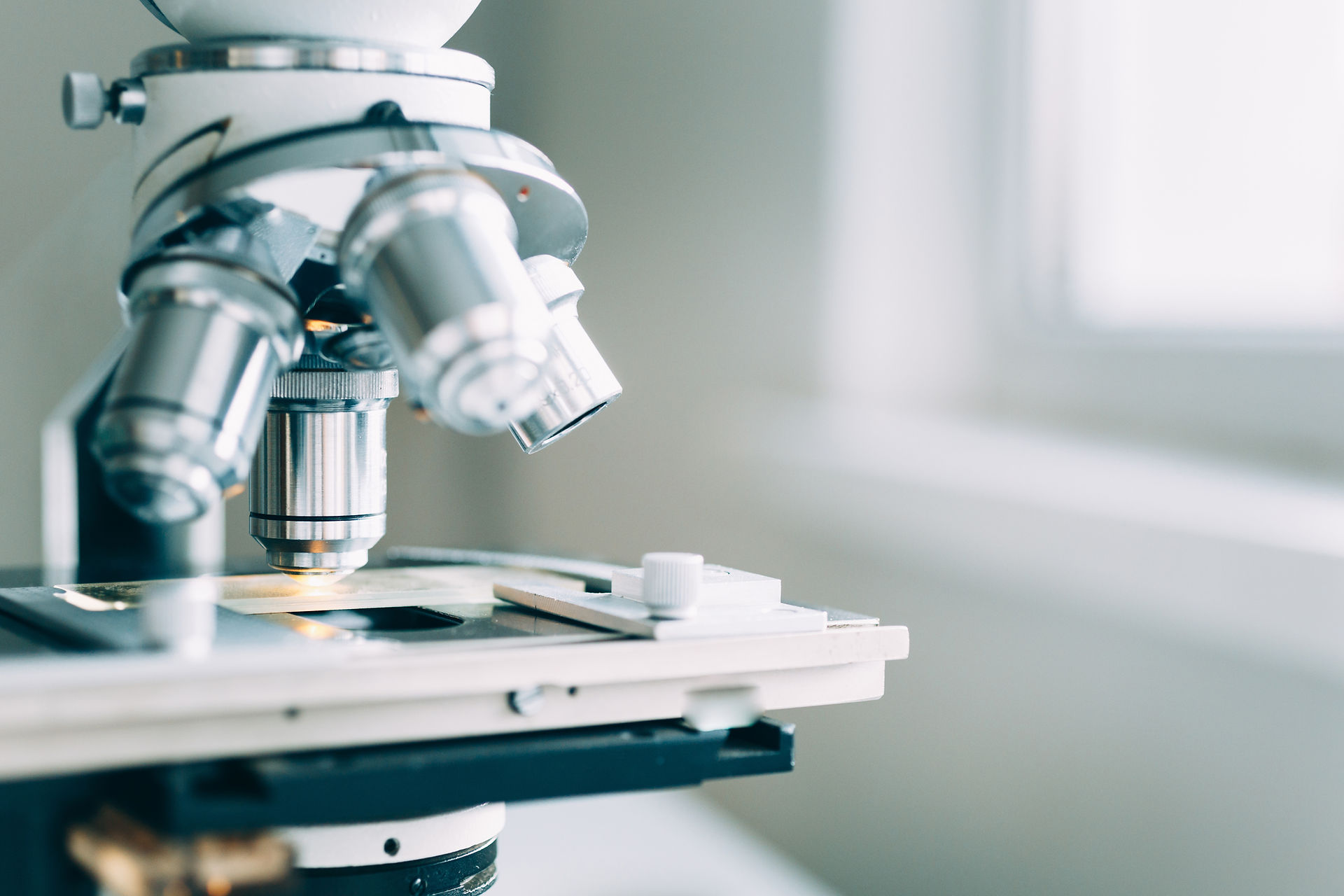
Vasopressin.org
Current projects.

Autonomic dysfunction plays a major role in neurogenic hypertension. We have used microarray gene profiling to generate catalogues of the mRNAs expressed in brain regions involved in regulating cardiovascular parameters in the adult male spontaneously hypertensive rat (SHR) and its normotensive Wistar-Kyoto (WKY) parent. Comparison of these lists has enabled us to identify genes that are expressed differentially between WKY and SHR. We hypothesise that hypertension is associated with changes in the expression of a network of a large number of genes in the brain that, through their activity, form a functional network. Based on purely unbiased mathematical criteria, we have sought to describe this network, and we further hypothesise that disruption of the network is sufficient to restore normal cardiovascular balance to the organism, and that nodal genes may represent effective targets for therapeutic intervention in normalising arterial pressure.
The identification and function of genes contributing to neurogenic hypertension.

There is considerable anecdotal evidence that a sedentary lifestyle can lead to ill heath in old age, whereas physical activity has long-term health and wellbeing benefits. The reasons why homeostatic systems deteriorate in the elderly, and why exercise can ameliorate this deterioration, are not well understood. However, there is growing evidence that autonomic nervous system (ANS) imbalance, where sympathetic nervous system over-activity dominates over parasympathetic activity, is involved in a wide range of chronic conditions of old-age, such as obesity, insulin resistance, diabetes, heart failure, stroke and hypertension. Whilst exercise can help restore ANS balance, physiological understanding of this phenomenon is scant.
We hypothesise that the development and/or maintenance of the autonomic imbalance is associated with changes in the expression of a large number of genes in the brain that, through their activity, form one or more functional networks that can be influenced by both age and by lifestyle factors, such as physical activity. In order to test this hypothesis, we will:
• identify putative networks by carrying out detailed and comprehensive transcriptome analysis on brain regions involved in the regulation of autonomic ouflow. Expression will be compared in normal animals (WKY rats), and in animals with a genetic predisposition to autonomic imbalance characterised by the development of hypertension and loss of heart rate variability (SHRs).
• examine gene expression changes as animals age
• ask if gene expression is altered by physical exercise, initiated either before or after the onset of autonomic imbalance.
• use the latest bioinformatic tools to construct putative pathways and networks that may govern these processes, and we will identify nodal, or hub genes with many connections.
• test whether these hubs are important physiologically by altering the expression of these nodal genes using in vivo somatic gene transfer approaches. This will be followed by robust molecular, cellular and, importantly, physiological analysis, that will reveal the roles of these hub genes in network stability and their response to external cues.
Amelioration of the autonomic imbalances of old age with exercise - exploring the molecular and physiological mechanisms

The neuroendocrine reflexes regulating water balance are centred on hypothalamic magnocellular neurones that synthesise the antidiuretic peptide hormone vasopressin (AVP). These neurones send axonal projections to the posterior pituitary gland, from where AVP is released into the blood. As a consequence of the depletion of pituitary stores that accompanies chronic osmotic stimulation, there is a need to synthesise more AVP. As we showed 25 years ago, this starts with an increase in transcription, and results in an increase in the abundance of mature mRNAs. Considerable effort has been expended in the subsequent years attempting to define the molecular mechanism responsible, but they have remained largely elusive.
In order to overcome this problem, we decided to document the transcriptome of the rat hypothalamus, and described it changes following the osmotic stimuli dehydration or salt loading. On of the genes thus identified was basic leucine zipper transcription factor cAMP-responsive element binding protein 3 like 1 (CREB3L1, also called OASIS), which is robustly up-regulated in AVP neurones by hypersomostic cues. CREB3L1 is an endoplasmic reticulum (ER) and Golgi resident protein that is activated by regulated intramembrane proteolysis, resulting in the release of an N-terminal activating domain that is transported to the nucleus, where it regulates the transcription of target genes. We tested the hypothesis that CREB3L1 can activate AVP gene transcription. We showed that CREB3L1 induces the expression of rat AVP promoter-luciferase reporter constructs, and direct binding of CREB3L1 to the AVP promoter was shown by chromatin immunoprecipitation both in vitro and in the SON itself. Injection of a lentiviral vector expressing a constitutively active mutant form of CREB3L1 into rat SON and PVN increased AVP biosynthesis.
It is known that cAMP levels are increased in the SON and PVN following chronic osmotic stimulation, and it has been suggested that this intracellular signalling pathway maybe important in the up-regulation of AVP expression. We have now shown that CREB3L1 mRNA expression is up-regulated by cAMP pathways, and that shRNA knockdown of CREB3L1 blocks the cAMP-mediated activation of AVP expression. Interestingly, inhibition of protein synthesis blocks the cAMP-mediated up-regulation of CREB3L1 expression, suggesting the requirement for the synthesis of an intermediary “Mystery Transcription Factor”.
We are now using a multi-disciplinary combination of state-of-the-art epigenomic (ChIPseq), transcriptomic (RNAseq), and proteomic/neuropeptidomic (yeast two hybrid and mass spectrometry), technologies, combined with state-of-the-art bioinformatics, to decipher the molecular pathways by which CREB3L1 functions in hypothalamic neurones that control hydromineral homeostasis. In doing so, we will address 6 interrelated questions, the answers to which will reveal the detailed mechanisms CREB3L1 regulation and function, within the context of the brain mechanisms that regulate water balance:
Q1. What are the molecular mechanisms that mediate the regulation of Creb3l1 by physiological cues? What is the identity of the “Mystery Transcription Factor”.
Q2. What are the molecular interactions in the hypothalamus that facilitate CREB3L1 actions?
Q3. What genes does CREB3L1 regulate in the SON and PVN?
Q4. How does CREB3L1 affect neuropeptide biosynthesis in the HNS?
Q5 Can we construct and test in silico models of the integrated mechanisms of CREB3L1 function?
Q6 What are the physiological roles of CREB3L1 and interacting genes?
Regulatory and functional pathways mediating the control of central osmotic defences by hypothalamic transcription factor CREB3L1
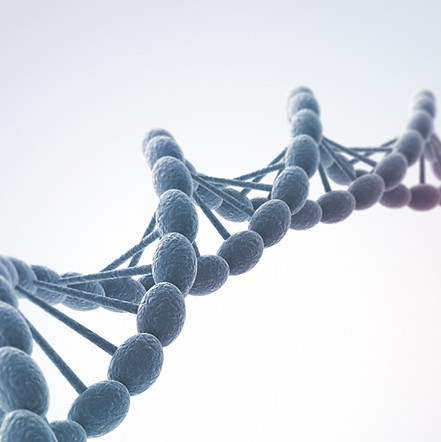
The precise regulation water balance is essential for survival and good health, and when threatened, osmotic stability is aggressively defended. However, these mechanisms go wrong in old age, and disorders of fluid balance are a frequent cause of morbidity and mortality in the elderly. The brain mechanisms responsible are located in hypothalamic magnocellular neurones, the axons of which terminate in the posterior pituitary gland. These neurones make the antidiuretic peptide hormone vasopressin (AVP), which acts at the level of the kidney to provoke water conservation. As a consequence of the depletion of pituitary stores that accompanies chronic osmotic stimulation, there is a need to synthesise more AVP. This starts with an increase in transcription, and results in an increase in the abundance of mature mRNAs. In addition, the AVP mRNA is subject to post-transcriptional modification in the form of an increase in the length of the 3' poly(A) tail. We documented the transcriptome of the rat hypothalamus, and described changes following dehydration or salt loading. One of the genes identified was RNA binding protein Caprin-2. We showed that Caprin-2 binds to the AVP mRNA, and lentiviral mediated snRNA knockdown of Caprin-2 in the osmotically stimulated hypothalamus shortens the AVP mRNA poly(A) tail and reduces transcript abundance. In an in vitro system, Caprin-2 over-expression enhanced the abundance and poly(A) tail length of the AVP mRNA. Importantly, Caprin-2 knock-down leads to dysfunction of the normal physiological response to salt loading, an osmotic challenge which in healthy rats leads to a gradual increase of urine output and fluid intake. Caprin-2 knockdown results in a significant decrease in urine output and fluid intake, and an increase in urine osmolality and plasma AVP levels. We have gone on to show that osmoregulatory dysfunction in aged rats is associated with both an increase in Caprin-2 expression and an increase in AVP mRNA poly(A) tail length. Mathematical analysis of our transcriptome data revealed that Caprin-2 is a central hub in a putative regulatory network. Importantly, knockdown or over-expression of three putative Caprin-2 targets - Pdyn, prodynorphin (Pdyn), Opsin 3 (Opn3) and b-haemoglobin (Hbb) - in vitro had opposite effects on target mRNA abundance. These data raise new questions regarding the molecular nature and physiological functions of the regulatory interactions in the Caprin-2 gene network. Importantly, it affords us the opportunity to deconstruct the network in vivo, and ask about the physiological consequences in both young and old animals. We will now ask about the molecular nature of the network interactions, how they impact on hormone elaboration and secretion, and ultimately how they control homeostasis. Comparing young and old animals, we are now using a multi-disciplinary combination of state-of-the-art transcriptomic (RNAseq and eCLIP) and proteomic (mass spectrometry) to address 10 interrelated questions, the answers to which will reveal the detailed mechanisms Caprin-2 network regulation and function within the context of the brain mechanisms that regulate water balance -
*Does Caprin-2 bind to target gene transcripts?
*Does Caprin-2 modulate the poly(A) tail length of target mRNAs?
*Does Caprin-2 binding and/or poly(A) tail extension affect mRNA translation?
*Is Caprin-2 part of an mRNP granule?
*What is the entire hypothalamic Caprin-2 binding mRNA binding repertoire?
*How does Caprin-2 affect the hypothalamic transcriptome?
*How does Caprin-2 affect neuropeptide biosynthesis in the hypothalamus?
*Can we construct in silco models of the entire Caprin-2 regulatory network?
*What are the physiological consequences of in vivo network deconstruction?
*Can transgenetic modulation of the Caprin-2 network restore osmoregulatory function in old age?
The role of hypothalamic RNA binding protein Caprin2 in osmoregulatory dysfunction in old age.
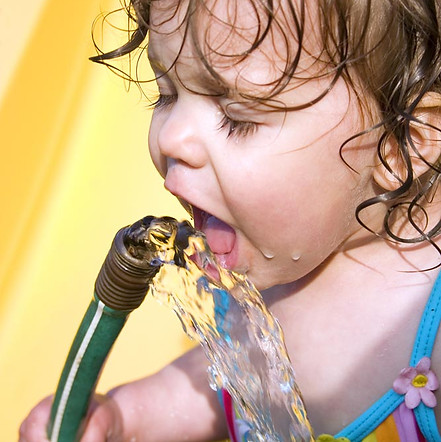
Over the past decade, it has become clear that mammalian genomes encode thousands of long non-coding RNAs (lncRNAs). Many long lncRNAs act in the nucleus to regulate gene expression, but their physiological functions remain to be determined. We aim to decipher the mechanisms whereby brain nuclear lncRNA Giot1 controls salt palatability and consumption.
The precise regulation water balance is essential for survival and good health and wellbeing, and when threatened, osmotic stability is aggressively defended. However, these mechanisms go wrong in old age, and diminished thirst and salt-appetite can result in disorders of fluid balance that are a frequent cause of morbidity and mortality in the elderly.
Integrated neuroendocrine and behavioural mechanisms function to control the excretion and consumption of water and salt in order to maintain the optimal bodily composition required for good health. Given a choice, rats would rather consume 0.9% w/v NaCl over water (appetite), but would rather consume water over 2% w/v NaCl (aversion). However, aversion is overcome if 2% w/v NaCl is the only fluid source (salt loading for up to 7 days, SL); the salty solution is avidly consumed. At the same time, integrative hypothalamic structures (the paraventricular nucleus, PVN, and the supraoptic nucleus, SON) activate the secretion of the antidiuretic neuropeptide hormone arginine vasopressin (VP) from magnocellular neuron (MCN) axon terminals in the posterior pituitary (PP), which acts on the kidney to provoke water conservation.
We have identified a nuclear lncRNA called Giot1 that is robustly up-regulated in the PVN and SON by SL. We have shown that:
*Giot1 activates salt drinking in SL animals.
*Giot1 regulates the expression of the gene encoding the endogenous opioid peptide dynorphin (Dyn).
*Blockade of Dyn kappa-opioid receptors (KORs) at the level of the amygdala (Amyg) can inhibit salt intake during SL.
*Expression of Giot1 increases in the PVN and SON with old age in rats.
We suggest that VP MCNs paradigmatic for the novel concept that neuroendocrine neurons can be involved in the simultaneous dual regulation of homeostasis and allostasis. In response to life-threatening physiological cues, these neurones respond by regulating both PP hormone secretion (homeostatic) and neuronal neurotransmitter secretion (allostatic regulation of preference). In the context of their role in allostasis, we hypothesise that lncRNA Giot1 regulates the expression of neurotransmitters that enable salt ingestion, through projections to limbic areas under different physiological conditions. We further hypothesise that these mechanisms alter in old age.
To robustly challenge these hypotheses, we are now characterising the Giot1 RNA at the molecular level, and are investigate the physiological and behavioural roles of Giot1. Our aims are to:
*determine which structures in the nucleus contain Giot1 RNA.
*ask whether the Giot1 RNA is trapped in the nucleus, or whether it shuttles between nucleus and cytoplasm.
*elucidate the Giot1 lncRNA interactome, and determine how it regulates gene expression.
*map the projections of Giot1-expressing MCNs.
*investigate the involvement of Giot1, Dyn and KOR in salt palatability.
*ask whether Giot1 has a role in salt hunger.
*determine the physiological and behavioural roles of specific MCN-limbic connections.
*ask about the role of Giot1 in disturbances of salt homeostasis in old age.
The role of nuclear non-coding RNA Giot1 in salt and water intake throughout the life-course.
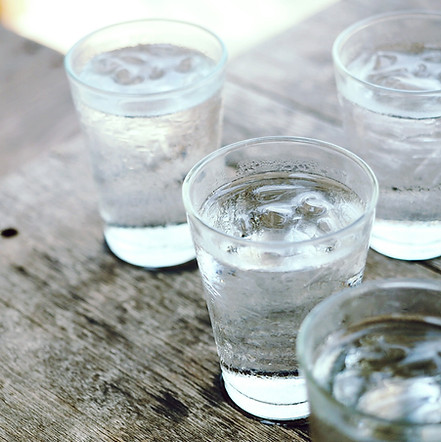
The brain mechanisms responsible for osmotic stability are located in the hypothalamo-neurohypophyseal system (HNS), which consists of magnocellular neurons located in the supraoptic nucleus (SON) and paraventicular nucleus (PVN), the axons of which terminate in the posterior pituitary gland. These neurones make the antidiuretic hormone vasopressin (AVP), which acts on the kidney to provoke water conservation. As a consequence of dehydration, the release of AVP from the hypothalamus is associated with many plastic changes that all work together to optimise hormone release and replenishment. These mechanisms go wrong in old age, and diminished thirst and salt-appetite can result in disorders of fluid balance that are a frequent cause of morbidity and mortality in the elderly.
We used microarrays to document the transcriptome of the rat hypothalamus, and described changes following the osmotic challenges of dehydration or salt loading. One of the genes identified as being robustly up-regulated in the supraoptic (SON) and paraventricular (PVN) nuclei of the hypothalamus following chronic dehydration for 3 days, and following consumption of 2% (w/v) NaCl (salt-loading, SL) was small G-protein Rasd1, a multifunctional intracellular signaling molecule with a role in numerous pathways governed by an extensive interactome that includes not only other signaling proteins, but also transcription factors. We have demonstrated that Rasd1 is a small G protein with a big role in the function related plasticity exhibited by the osmotically challenged hypothalamus. We have shown that in vivo knockdown of Rasd1 by viral-mediated delivery of shRNAs has no effect on water intake in euhydrated rats, but induced insatiable consumption of salt during SL as well as increased levels of circulating AVP. These data suggest that the role of Rasd1 is normally to inhibit these processes.
However, as Rasd1 is a multifunctional protein with an extensive interactome, the exact pathways involved still remain to be deciphered. We thus hypothesise that Rasd1 has a unique interactome in the HNS, and that Rasd1 binding proteins and Rasd regulated genes mediate diverse vital physiological functions that cooperatively contribute to osmotic defense mechanisms. We will thus explore the interactions between Rasd1 and partner proteins, and Rasd1 and the genome, within the context of HNS osmoregulatory plasticity. Our specific objectives are as follows:
*In the different compartments of the HNS, we will determine whether Rasd1 forms complexes with proteins previously described as being part of the Rasd1 interactome.
*Two unbiased methodologies will define the entirety of the Rasd1 interactome in different compartments of the HNS, thus confirming previously described interactions, as well as identifying new ones. This information will place Rasd1 into functional pathways that can be tested physiologically in vivo.
*We will identify genes regulated by transcription factors that complex with Rasd1.
*A major bottleneck of the “Omic” era is the sheer scale and complexity of the datasets, and the resulting daunting problem of identifying suitable targets for often expensive and time consuming physiological studies. Rather than “cherry-pick” our data, we will use unbiased mathematical criteria to select genes worthy of functional study.
*It is important to confirm expression of interacting protein and Rasd1-regulated genes in Rasd1- and AVP-expressing cells.
*We will dissect the role of interacting genes and proteins in overall physiological role of Rasd1. These studies are a necessary prelude to future molecular and cellular mechanistic studies, particularly in regard to the ageing process.
Deciphering the physiological roles of the Rasd1 interactome in the central control of hydromineral homeostasis.
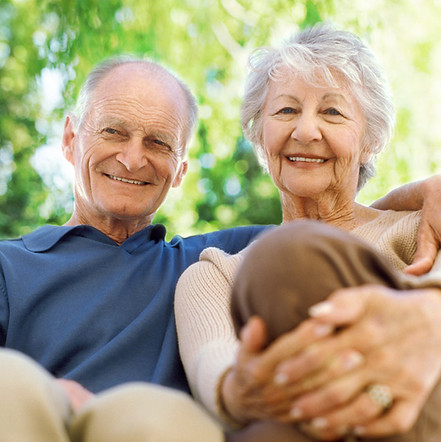
Age and gender are significant risk factors in the development of hypertension, a major cause of morbidity and mortality in worldwide. Blood pressure (BP) is generally lower in pre-menopausal women compared to age-matched males, and younger men are at greater risk of cardiovascular disease than pre-menopausal women of the same age. However, whilst age is a contributory factor in the development of hypertension in both men and women, the onset of menopause increases hugely the risk of developing high BP in women, such that the prevalence of hypertension in post-menopausal women exceeds that of their male peers. This project is based on the compelling evidence that alterations in the balance of cardiovascular autonomic motor outflow and consequent over-activity of the sympathetic nervous system contributes to essential hypertension (neurogenic hypertension). Indeed, in essential hypertension homeostatic reflexes that regulate arterial pressure are re-set to higher levels due to alterations in central nervous system circuits. Ultimately, the raised BP increases the risk of coronary heart disease, renal failure, stroke and end organ damage. We propose that these changes can be described in terms of altered patterns of gene expression within these central nervous cardiovascular circuits. Knowing more about these genes has considerable therapeutic implications. Specifically, we hypothesise that the expression of genes in key brainstem and hypothalamic structures is different in:
- young and old males, and that these differences contribute to the progressive worsening of the symptoms of hypertension.
- age-matched male and females, and that these differences contribute to the gender-related development of hypertension.
- pre- and post-menopausal females, with and without intact ovaries, and that these differences contribute to the development of post-menopausal hypertension, which is dependent upon ovarian factors in younger life.
We will test these hypotheses by comparing normal rats with rats genetically predisposed to develop hypertension. We will interrogate microarrays with targets derived from hypothalamic and brainstem regions known to be important in cardiovascular control. By comparing male and female, young and old, and intact and ovariectomised animals, we will identify gene expression signatures that define, and may mediate, age- and gender-related differences in the prevalence of hypertension.
Physiological genomics of gender and age differences in neurogenic hypertension.

The one-humped Arabian camel (Camelus dromedarius) has a remarkable capacity to survive extended periods of water deprivation. Whereas other mammals would die from circulatory failure when water loss reaches 12% of body weight, the camel can tolerate up to 30%. This is achieved by minimal evaporative cooling, low urinary output, water extraction from undigested food residues, and an increase in body temperature from 34C to 42C. The mammalian brain regulates water balance through the synthesis and secretion of the antidiuretic hormone vasopressin (VP) by the hypothalamo-neurohypophyseal system (HNS). VP is synthesised in HNS neurones which project axons to terminals located in the neural lobe (NL) of the pituitary, where mature VP is stored until released into the circulation in response to electrical activity evoked by physiological cues. VP acts on the kidney to provoke water reabsorption. Interestingly, the ultrastructure of the camel HNS changes according to season, suggesting that in the arid conditions of summer, the dromedary’s HNS is in a state of permanent activation, in preparation for the likely prospect of water deprivation. We have recently sequenced the genome of Camelus dromedarius. Based on this unique resource, we are now using a multi-disciplinary functional genomic approach combining state-of-the-art transcriptomic (RNAseq) and proteomic technologies to comprehensively describe, at the molecular level, the seasonal adaptations of the camel brain, and the responses to chronic dehydration. Camels will be subjected to comprehensive physiological and morphological investigation. HNS transcriptome and neuropeptidome datasets will be compared to existing rodent data sets, and subject to bioinformatic analyses to reveal nodal target genes. The functions of prioritized gene targets will be tested in vivo in rodent established models by HNS injection of viral vectors that over-express or knockdown target genes.
Functional genomics of the seasonal responses of the camel hypothalamo-neurohypophysial system to dehydration: anticipation and adaptation.
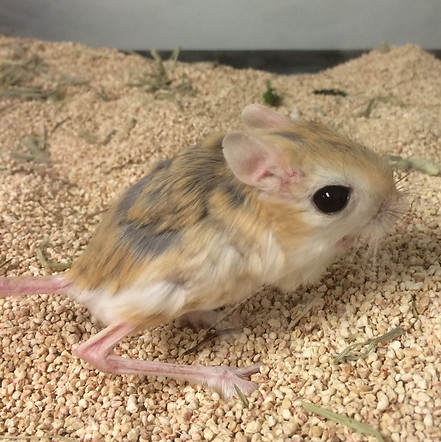
Whilst water balance is aggressively defended in all terrestrial animals, this is all the more so in those species (xerocoles) that thrive in the hot, arid conditions of the Arabian and North African deserts. The harsh environment of the desert is host to a remarkably diverse spectrum of adapted mammals, from the magnificent one-humped Arabian dromedary camel (Camelus dromedarius), to tiny rodents, such as the Lesser Egyptian Jerboa (Jaculus jaculus). In order to understand the physiological mechanisms that enable mammals to survive in arid regions, which, in turn, will teach us about biological adaptations to climate change, in this proposal we focus on the latter, more convenient, rodent model,
Jerboas do not drink liquids as such, deriving all of their water from food, and they are able to survive prolonged periods of dehydration. Water economy is thus vital and, this is achieved by the production of a low volume of highly concentrated urine, as a consequence of the highly efficient reabsorption of water at the level of the kidney. This is mediated by the actions of the antidiuretic hormone arginine vasopressin (AVP), which is made in a specialised part of the brain called the hypothalamo-neurohypophysial system (HNS).
The HNS consists of the large peptidergic magnocellular neurones (MCNs) of the hypothalamic supraoptic (SON) and paraventricular (PVN) nuclei, the axons of which course though the internal zone of the median eminence (ME) to terminations on blood capillaries of the posterior pituitary (PP) gland, a neuro-vascular interface through which the brain regulates peripheral organs in order to maintain homeostasis. The rise in plasma osmolality that follows dehydration is detected by intrinsic MCN mechanisms and by specialised osmoreceptive neurons in the circumventricular organs (CVOs) such as the subfornical organ (SFO), which provide excitatory inputs to shape the firing activity of MCNs for AVP secretion. Upon release, AVP travels through the blood to specific kidney receptors where it promotes water reabsorption in the collecting duct.
Dehydration evokes a dramatic functional remodelling of the HNS, a process known as function-related plasticity. A plethora of activity-dependent changes in the morphology, electrical properties and biosynthetic and secretory activity of the HNS have been described, all of which might contribute to the facilitation of hormone production and delivery, and hence survival. We sought to understand this plasticity in terms of changes in global gene expression patterns. Thus, we used Affymetrix GeneChips, and latterly RNAseq, to document the transcriptomes of the rat and the mouse HNS, and to describe how these change following dehydration. Bioinformatic analysis was then used to identify nodal target genes, the functions of which were then investigated in vivo. We have developed viral gene deliver methods that enable us to manipulate the activity of specific endogenous genes within the HNS of the intact conscious rats. We can ask how this affect the physiology of the rat in terms of its response to dehydrating cues.
We are applying this successful strategy to Jaculus jaculus in order to understand the physiological mechanisms that enable this species to survive in the desert without drinking. We will describe, at the molecular level, the response of the Jaculus jaculus HNS to chronic dehydration. Animals will be subjected to comprehensive physiological, morphological, transcriptomic and neuropeptidomic investigation. Datasets will be subject to bioinformatic analyses that will reveal target genes, the functions of which will be tested in vivo in our established rat models.